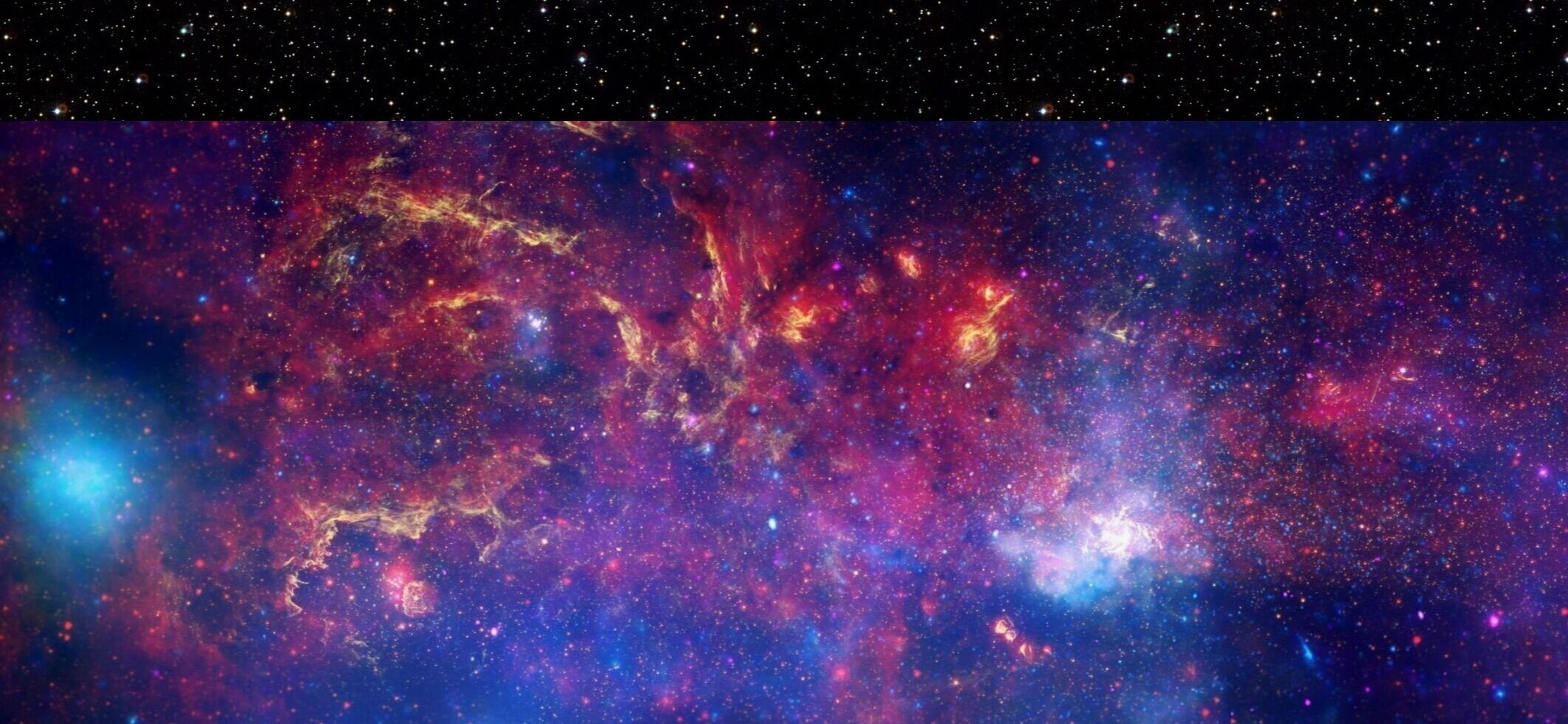
The Universe is out there, waiting for you to discover it.
There’s a cosmic story uniting us.
We’re determined to bring it to everyone.
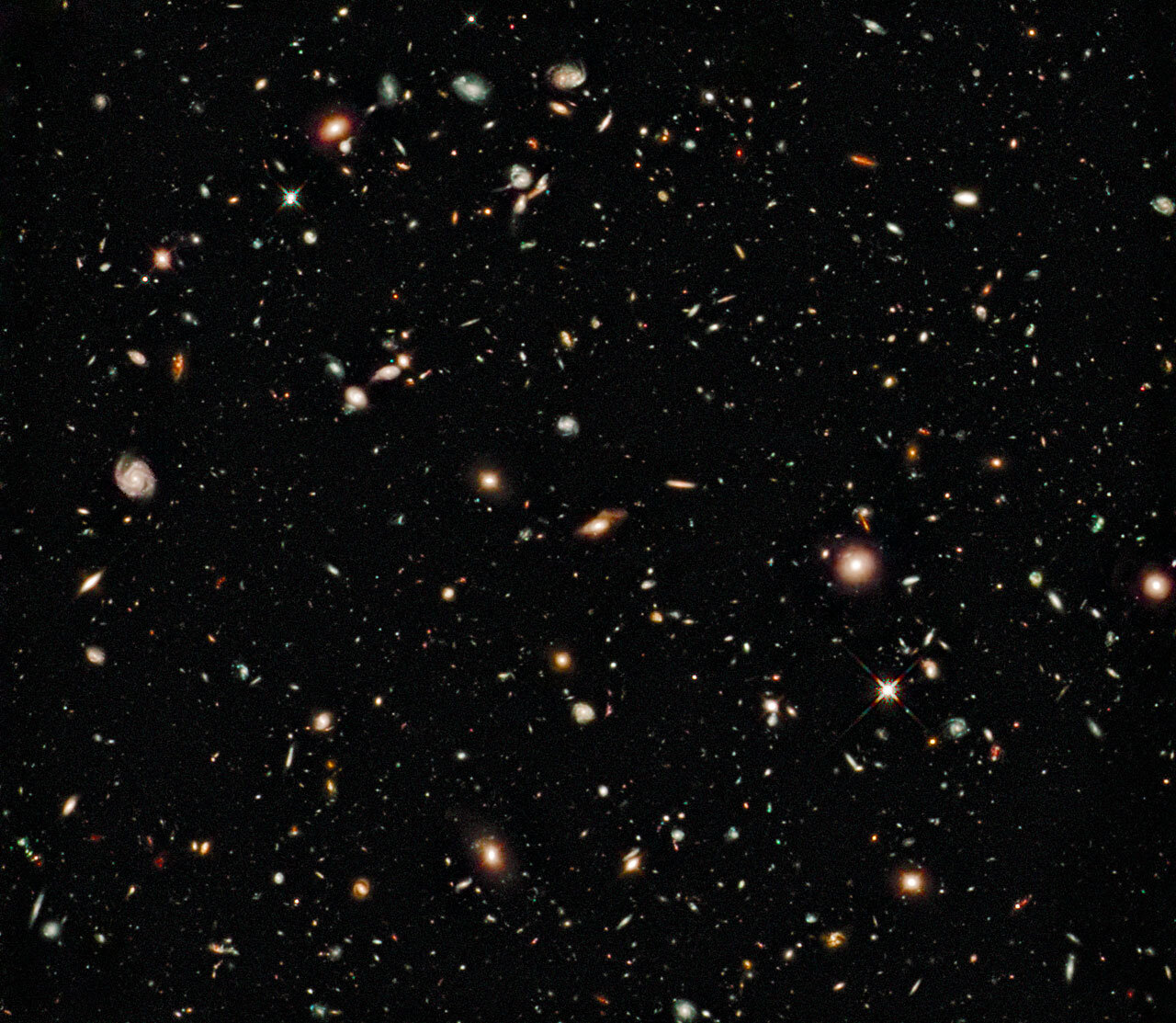
Our Creations
On Big Think, Medium, and Patreon
From before the Big Bang to our ultimate cosmic fate
Exploring the Universe on SoundCloud, iTunes, and more
Get early access, exclusive content, and bonus rewards
The Universe tells a story
we all deserve to hear
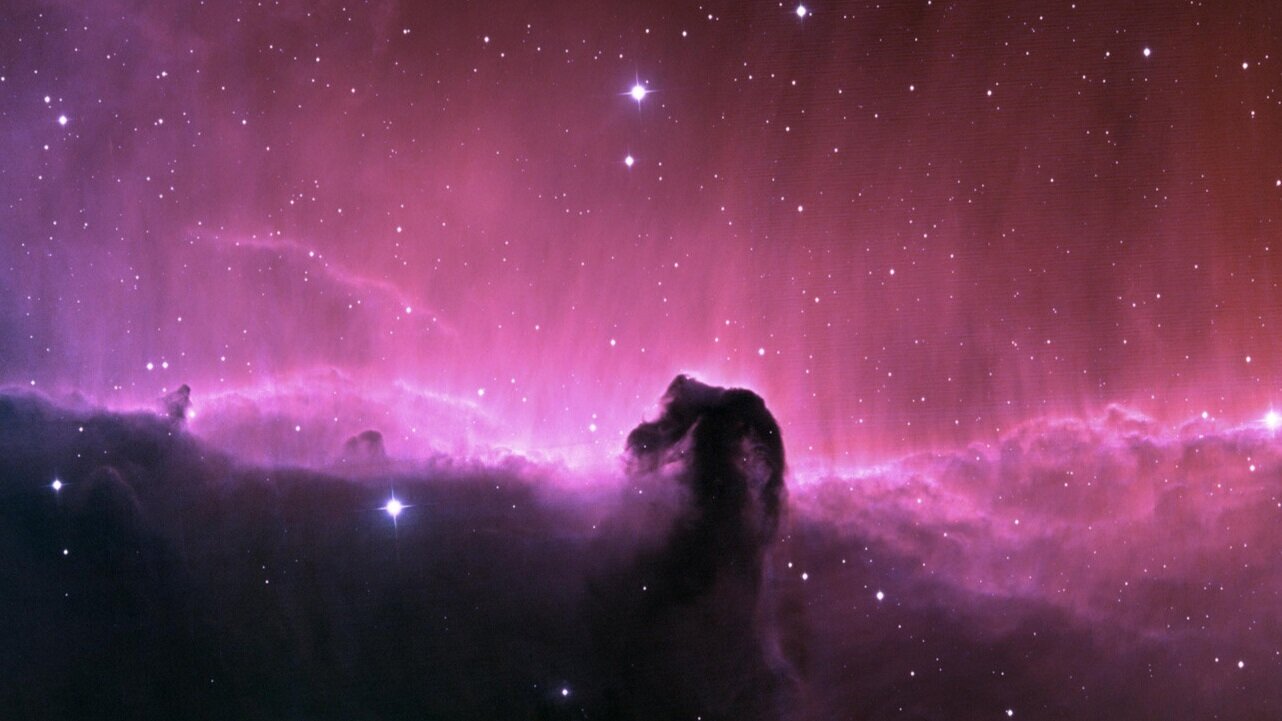
Want to know what this Universe is, how it was born, how it grew to be this way, and what its ultimate fate is?
So do we. Let’s take that journey together.
Learn more about our mission, history, and goals.
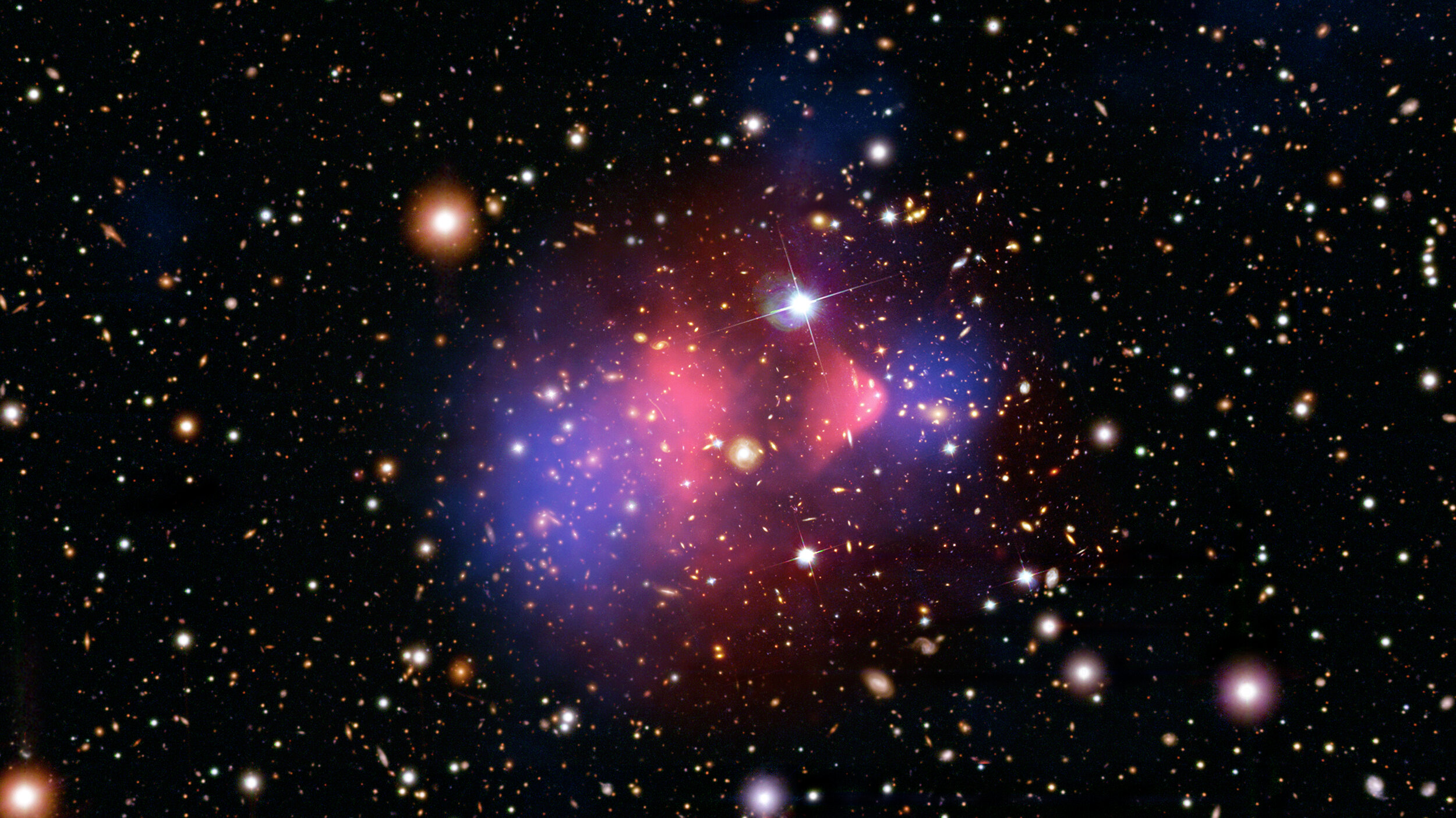
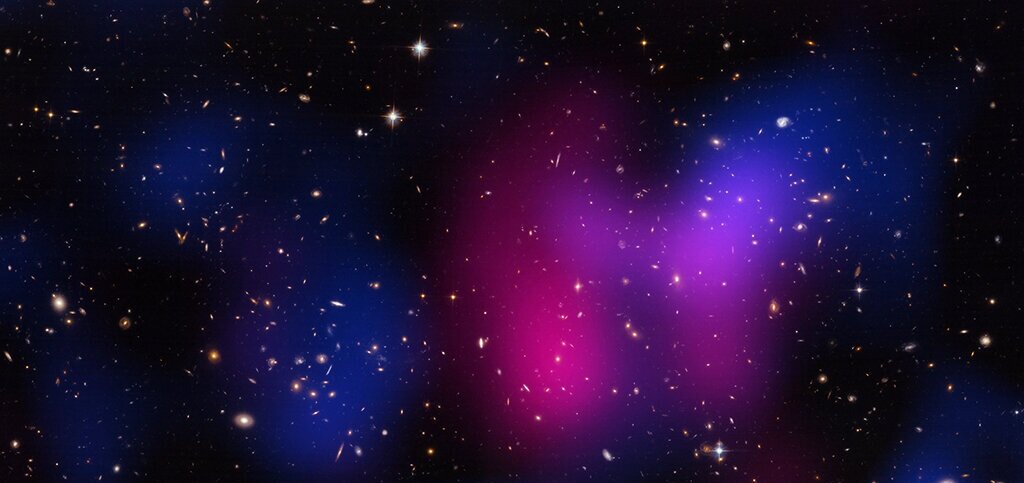
Contact
The Universe is yours to explore.
We’re here to bring it to you.